
![]() | ![]() | ![]() | ![]() |
---|---|---|---|
![]() |
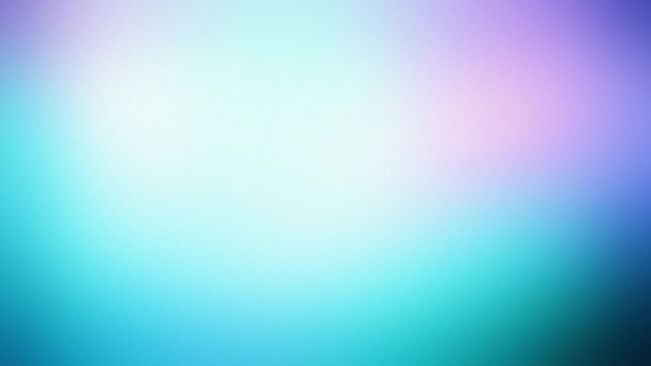
Intro : Definition
What is the Albedo effect
Albedo or reflection coefficient, derived from Latin albedo "whiteness" (or reflected sunlight) in turn from albus "white", is the diffuse reflectivity or reflecting power of a surface.
Astronomers define the reflectivity of an object in space using a term called albedo.
This is the amount of electromagnetic radiation that reflects away, compared to the amount that gets absorbed.
A perfectly reflective surface would get an albedo score of 1, while a completely dark object would have an albedo of 0. Of course, it’s not that black and white in nature, and all objects have an albedo score that ranges between 0 and 1.
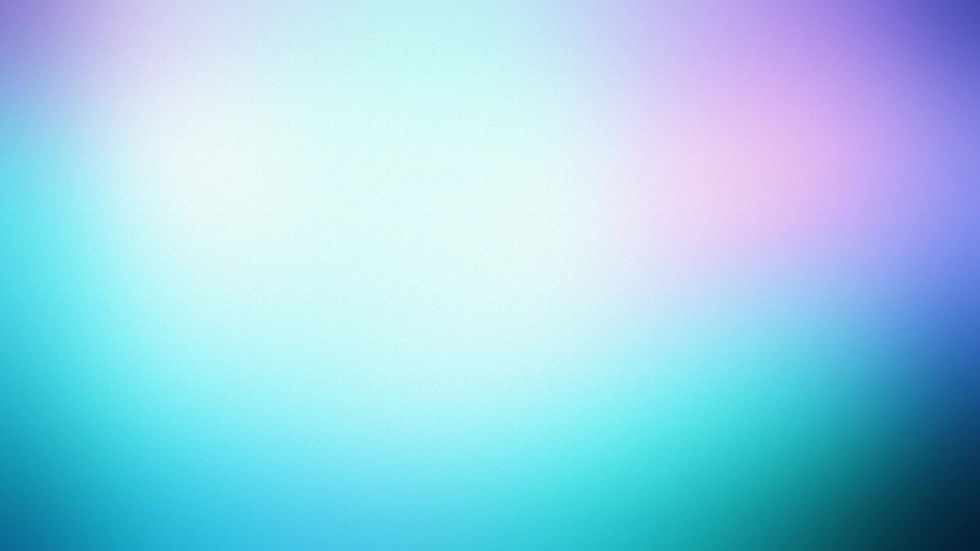
Intro : Definition
Why is the Albedo effect
important
Here on Earth, the albedo effect has a significant impact on our climate.
The lower the albedo, the more radiation from the Sun that gets absorbed by the planet and temperatures will rise.
If the albedo is higher, and the Earth is more reflective, more of the radiation is returned to space, and the planet cools.
The more radiation reflected the less global warming that occurs.
You have probably heard how the ice caps reflect solar radiation and hence why their melting is such a big issue. Fresh snow has an albedo of approximately 0.9.
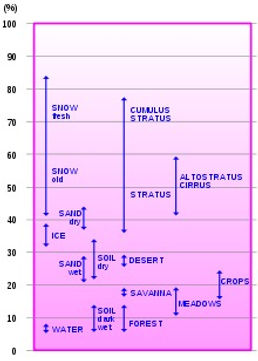

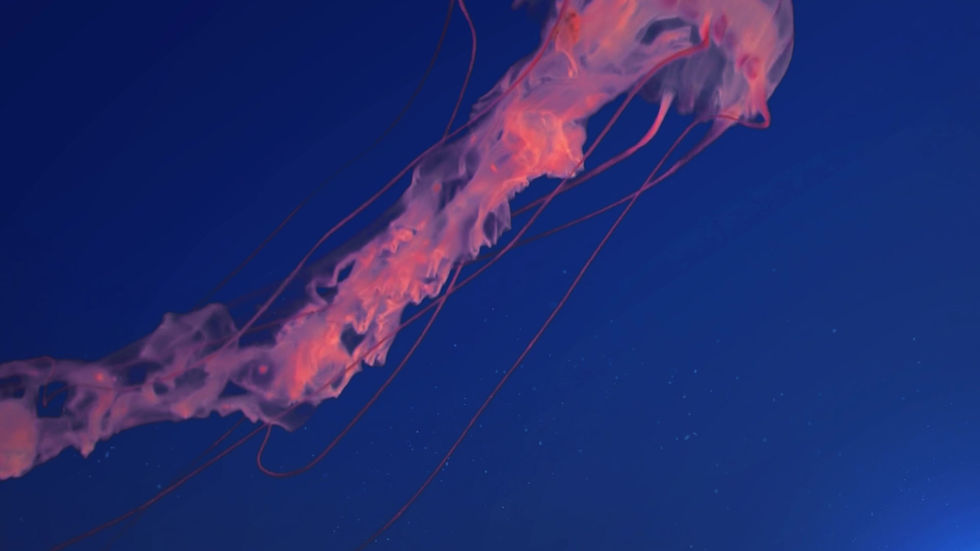
Introduction
여기를 클릭해 내용을 입력하세요. 마우스로 텍스트 상자의 위치와 크기를 변경하고, 텍스트 에디터에서 글꼴과 색상을 선택해 보세요. 다양한 한글 글꼴을 사용하려면, 글꼴 언어를 한국어로 지정하세요. 사용자 친화적인 드래그-앤-드롭 방식의 홈페이지 제작 도구를 사용해 코딩없이 홈페이지를 만들어보세요. 간단한 조작으로 데스크톱 홈페이지를 모바일 기기에 최적화 할수도 있습니다. 홈페이지를 게시하기전, 방문자들의 입장에서 홈페이지를 미리 사용해보세요. '미리보기' 메뉴를 통해 홈페이지를 게시하기전에 방문자들이 사용하는 그대로 홈페이지의 기능을 시험해볼수 있습니다. 전세계 5000만명 이상이 홈페이지 제작을 위해 Wix를 선택한 이유
를 확인해보세요.
Measuring Albedo
NASA's Clouds and the Earth's Radiant Energy System, or CERES
Earth's surface albedo is regularly estimated via Earth observation satellite sensors such as NASA's MODIS instruments on board the Terra and Aqua satellites.
As the total amount of reflected radiation cannot be directly measured by satellite, a mathematical model of the BRDF is used to translate a sample set of satellite reflectance measurements into estimates of directional-hemispherical reflectance and bi-hemispherical reflectance.
Earth's surface albedo is regularly estimated via Earth observation satellite sensors such as NASA's MODIS instruments on board the Terra and Aqua satellites.
As the total amount of reflected radiation cannot be directly measured by satellite, a mathematical model of the BRDF is used to translate a sample set of satellite reflectance measurements into estimates of directional-hemispherical reflectance and bi-hemispherical reflectance.
Earth's surface albedo is regularly estimated via Earth observation satellite sensors such as NASA's MODIS instruments on board the Terra and Aqua satellites.
As the total amount of reflected radiation cannot be directly measured by satellite, a mathematical model of the BRDF is used to translate a sample set of satellite reflectance measurements into estimates of directional-hemispherical reflectance and bi-hemispherical reflectance.
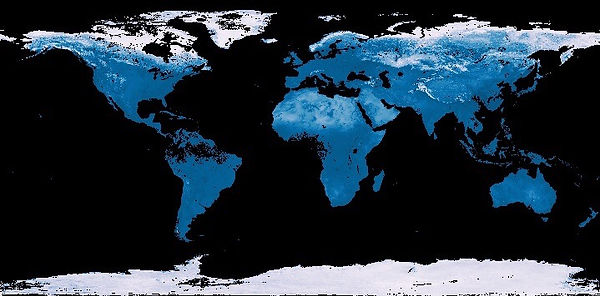
(http://neo.sci.gsfc.nasa.gov/view.php?datasetId=MCD43C3_M_BSA NASA.Albedo observation)
Scientists have been examining this balance sheet with a series of space-based sensors known as Clouds and the Earth’s Radiant Energy System, or CERES. The instruments use scanning radiometers to measure both the shortwave solar energy reflected by the planet (albedo) and the longwave thermal energy emitted by it. The first CERES went into space in 1997 on the Tropical Rainfall Measuring Mission, and three more have gone up on Terra, Aqua, and Suomi-NPP. The last remaining CERES instrument will fly on the JPSS-1 satellite, and a follow-on, the Radiation Budget Instrument (RBI), will fly on JPSS-2.
If Earth was completely covered in ice, its albedo would be about 0.84, meaning it would reflect most (84 percent) of the sunlight that hit it. On the other hand, if Earth was covered by a dark green forest canopy, the albedo would be about 0.14 (most of the sunlight would get absorbed). Changes in ice cover, cloudiness, airborne pollution, or land cover (from forest to farmland, for instance) all have subtle effects on global albedo. Using satellite measurements accumulated since the late 1970s, scientists estimate Earth’s average albedo is about 0.30.
The maps above show how the reflectivity of Earth—the amount of sunlight reflected back into space—changed between March 1, 2000, and December 31, 2011. This global picture of reflectivity (also called albedo) appears to be a muddle, with different areas reflecting more or less sunlight over the 12-year record. Shades of blue mark areas that reflected more sunlight over time (increasing albedo), and orange areas denote less reflection (lower albedo).
Taken across the planet, no significant global trend appears. As noted in the anomaly plot below, global albedo rose and fell in different years, but did not necessarily head in either direction for long.
In the maps at the top of the page, however, some regional patterns emerge. At the North Pole, reflectivity decreased markedly, a result of the declining sea ice on the Arctic Ocean and increasing dust and soot on top of the ice. Around the South Pole, reflectivity is down around West Antarctica and up slightly in parts of East Antarctica, but there is no net gain or loss. At the same time, Antarctic sea ice there has been increasing slightly each year.
In the early 2000s, after the first few years of Terra-CERES measurements, it appeared that Earth’s albedo was declining, a phenomenon that was widely reported in scientific journals and on NASA Earth Observatory. But as more years of data accumulated, and as scientists began to better understand the data, they found that albedo was neither increasing nor declining over time. It was fluctuating a lot by year, though.
The result of the long time observation by CERES
“What the results show is that even at global scales, Earth’s albedo fluctuates markedly over short time periods due to natural variations in the climate system,” said Norman Loeb, CERES principal investigator at NASA’s Langley Research Center. Ice cover, cloud cover, and the amount of airborne particles—aerosols from pollution, volcanoes, and dust storms—can change reflectivity on scales from days to years. “We should not get fooled by short-term fluctuations in the data, as a longer record may reverse any short-term trend.”
“The results also suggest that in order to confidently detect changes in Earth’s albedo above natural variability, a much longer record is needed,” Loeb added. “It is paramount that we continue the CERES Terra, Aqua, and Suomi-NPP observations as long as possible, and launch follow-on Earth radiation budget instruments to ensure continued coverage of this fundamental property of the climate system.”
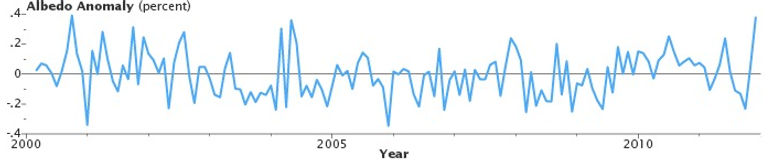
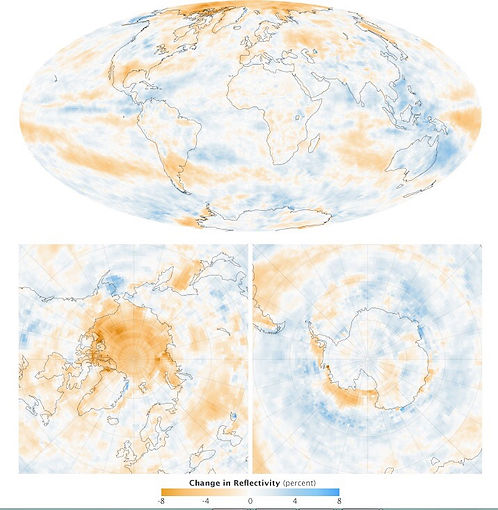
acquired March 1, 2000-December 31, 2011
Earth's surface albedo is regularly estimated via Earth observation satellite sensors such as NASA's MODIS instruments on board the Terra and Aqua satellites.
As the total amount of reflected radiation cannot be directly measured by satellite, a mathematical model of the BRDF is used to translate a sample set of satellite reflectance measurements into estimates of directional-hemispherical reflectance and bi-hemispherical reflectance.
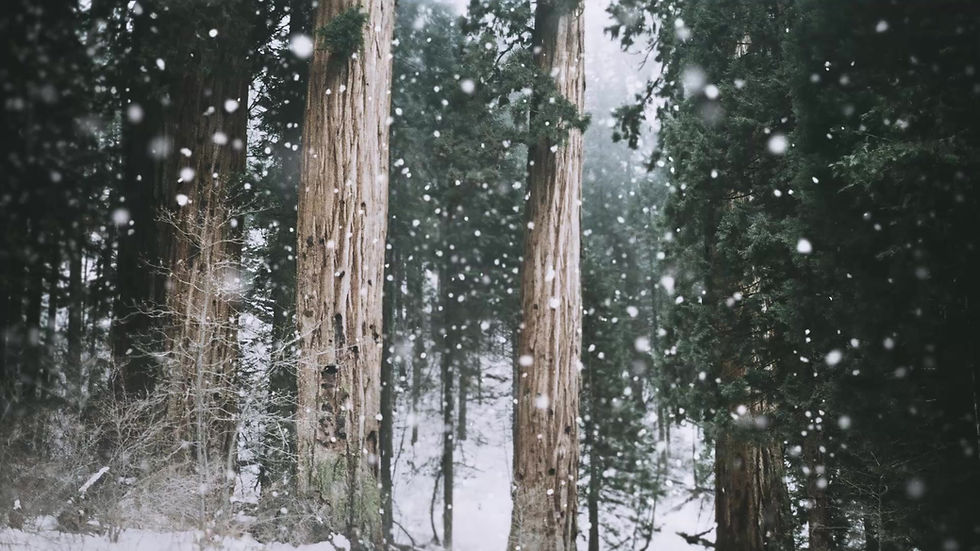
About Albedo
Terrestrial Albedo
Earth’s average Albedo
Albedo of typical materials in visible light range from up to 0.9 for fresh snow to about 0.04 for charcoal, one of the darkest substances. Deeply shadowed cavities can achieve an effective albedo approaching the zero of a black body. When seen from a distance, the ocean surface has a low albedo, as do most forests, whereas desert areas have some of the highest albedos among landforms. Most land areas are in an albedo range of 0.1 to 0.4. The average albedo of Earth is about 0.3.This is far higher than for the ocean primarily because of the contribution of clouds.
Earth’s average surface temperature
Earth's average surface temperature due to its albedo and the greenhouse effect is currently about 15 °C. If Earth were frozen entirely (and hence be more reflective) the average temperature of the planet would drop below −40 °C. If only the continental land masses became covered by glaciers, the mean temperature of the planet would drop to about 0 °C. In contrast, if the entire Earth is covered by water—a so-called aqua planet—the average temperature on the planet would rise to just under 27 °C.
(5-1)Factors of terrestrial albedo effect
VEGETATION
Because forests generally have a low albedo, (the majority of the ultraviolet and visible spectrum is absorbed through photosynthesis), some scientists have suggested that greater heat absorption by trees could offset some of the carbon benefits of afforestation (or offset the negative climate impacts of deforestation). In the case of evergreen forests with seasonal snow cover albedo reduction may be great enough for deforestation to cause a net cooling effect. Trees also impact climate in extremely complicated ways through evapotranspiration. The water vapor causes cooling on the land surface, causes heating where it condenses, acts a strong greenhouse gas, and can increase albedo when it condenses into clouds Scientists generally treat evapotranspiration as a net cooling impact, and the net climate impact of albedo and evapotranspiration changes from deforestation depends greatly on local climate.
In seasonally snow-covered zones, winter albedos of treeless areas are 10% to 50% higher than nearby forested areas because snow does not cover the trees as readily. Deciduous trees have an albedo value of about 0.15 to 0.18 whereas coniferous trees have a value of about 0.09 to 0.15.
Studies by the Hadley Centre have investigated the relative (generally warming) effect of albedo change and (cooling) effect of carbon sequestration on planting forests. They found that new forests in tropical and mid latitude areas tended to cool; new forests in high latitudes (e.g. Siberia) were neutral or perhaps warming.
SNOW
Snow albedo is highly variable, ranging from as high as 0.9 for freshly fallen snow, to about 0.4 for melting snow, and as low as 0.2 for dirty snow. Over Antarctica they average a little more than 0.8. If a marginally snow-covered area warms, snow tends to melt, lowering the albedo, and hence leading to more snowmelt because more radiation is being absorbed by the snowpack (the ice–albedo positive feedback). Cryoconite, powdery windblown dust containing soot, sometimes reduces albedo on glaciers and ice sheets. Hence, small errors in albedo can lead to large errors in energy estimates, which is why it is important to measure the albedo of snow-covered areas through remote sensing techniques rather than applying a single value over broad regions.
WATER
Water reflects light very differently from typical terrestrial materials. The reflectivity of a water surface is calculated using the Fresnel equations (see graph).
Reflectivity of smooth water at 20 °C (refractive index=1.333)
At the scale of the wavelength of light even wavy water is always smooth so the light is reflected in a locally specular manner (not diffusely). The glint of light off water is a commonplace effect of this. At small angles of incident light, waviness results in reduced reflectivity because of the steepness of the reflectivity-vs.-incident-angle curve and a locally increased average incident angle.
Although the reflectivity of water is very low at low and medium angles of incident light, it becomes very high at high angles of incident light such as those that occur on the illuminated side of Earth near the terminator (early morning, late afternoon, and near the poles). However, as mentioned above, waviness causes an appreciable reduction. Because light specularly reflected from water does not usually reach the viewer, water is usually considered to have a very low albedo in spite of its high reflectivity at high angles of incident light.
Note that white caps on waves look white (and have high albedo) because the water is foamed up, so there are many superimposed bubble surfaces which reflect, adding up their reflectivities. Fresh 'black' ice exhibits Fresnel reflection.
CLOUD
Cloud albedo has substantial influence over atmospheric temperatures. Different types of clouds exhibit different reflectivity, theoretically ranging in albedo from a minimum of near 0 to a maximum approaching 0.8.
"On any given day, about half of Earth is covered by clouds, which reflect more sunlight than land and water. Clouds keep Earth cool by reflecting sunlight, but they can also serve as blankets to trap warmth."
Clouds reflect more light back to space than does a cloudless blue sky. A cloud's albedo depends on several factors, including the cloud's height, its size, and the number and size of droplets inside the cloud. Clouds range in color from bright white to dark grey, because of the water droplets scattering light; more drops as well as bigger drops have a larger surface area and reflect more light. A large cumulonimbus cloud casts a dark shadow because light does not go through it easily. From space this same cloud would look bright white because it has a high albedo. A cirrus cloud on the other hand is nearly transparent but seems greyer from the space because its albedo is lower.
AEROSOL
Aerosols (very fine particles/droplets in the atmosphere) have both direct and indirect effects on Earth's radiative balance. The direct (albedo) effect is generally to cool the planet; the indirect effect (the particles act as cloud condensation nuclei and thereby change cloud properties) is less certain. As per the effects are:
Aerosol direct effect. Aerosols directly scatter and absorb radiation. The scattering of radiation causes atmospheric cooling, whereas absorption can cause atmospheric warming.
Aerosol indirect effect. Aerosols modify the properties of clouds through a subset of the aerosol population called cloud condensation nuclei. Increased nuclei concentrations lead to increased cloud droplet number concentrations, which in turn leads to increased cloud albedo, increased light scattering and radiative cooling (first indirect effect), but also leads to reduced precipitation efficiency and increased lifetime of the cloud (second indirect effect).
HUMAN ACTIVITY
Human activities (e.g. deforestation, farming, and urbanization) change the albedo of various areas around the globe. However, quantification of this effect on the global scale is difficult.
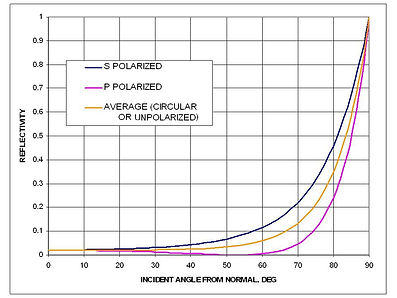